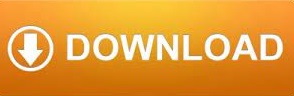
When the n +p junction of the switch is forward-biased, a large number of electrons will be injected to the p region of the SiGe waveguide causing an increase of carrier concentration. It is well known that the refractive index of SiGe is related to its carrier concentration. The operating principle of the switch is based on the total internal reflection caused by the plasma dispersion effect of the SiGe material. Lateral confinement is provided by a SiGe/SiO 2 ridge wall and the effective index induced by its geometry. In the ridge waveguide, an impurity-induced index step at the p-SiGe/p-Si interface is used for vertical confinement of input light. Figure 2.34c shows the cross-section view of the ridge waveguide layer structure. In the optimum design, two input intersecting angles are θ = 1.5° and one output branching angle is 2θ = 3°. The effective index method is used to analyze the behavior of the mode propagation and to determine the branch angle of the switch. The etching depth of all the ridge waveguides is designed to be 1.1 ± 0.1 μm for a Ge content of 4%. For design of all ridge waveguides, a thickness of 2.5 μm and width of 10 μm are chosen. The switches are designed for Si-based SiGe material with raised ridge waveguide structures for the single-mode operation. A schematic diagram of a photonic switch: (a) top view, (b) enlarged central section view, and (c) waveguide cross-section view.

Such a state is called a mirror-OFF state.Ģ.34. On the other hand, when no forward-bias voltage is applied, the refractive index of the material remains unchanged and there is no reflecting interface formed. As a result, the refractive index of the material will decrease in the region and a reflecting interface will be formed. If a forward-bias voltage is applied to the carrier injection region, the carrier concentration will increase. Each of the mirrors has two states, i.e., mirror-ON and mirror-OFF. mirror I’ and mirror II’, are actuated by applying forward-bias voltage. As the figure shows, the two carrier injection regions, I and II, are arranged in such a way that they can be controlled individually to switch optical signals from the three input ports to the two output ports. Figure 2.34b shows an enlarged central section with two electronically controlled carrier injection regions, which is basically an n +p junction. The central section is an intersecting part, which is the key part of this photonic switch. The input section consists of one straight waveguide 1 and two S-shaped waveguides 2 and 3 while output section consists of two S-shaped waveguides 4 and 5. It consists of an input section, a central section, and an output section.
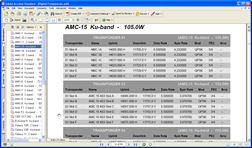
Figure 2.34a shows a schematic diagram of the proposed 3 × 2 switch with a multi-wavelength cross-connect structure 24.
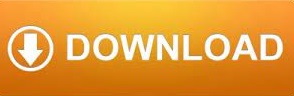